"It
has not escaped our notice that the specific pairing
we have postulated immediately suggests a possible
copying mechanism for the genetic material."
--
J.
Watson & F. Crick, 1953
|
- What Must the Genetic Material Be Able To Do?
As we will see over the next few units, the base pairing
proposed by Watson and Crick turned out to be very important
in the work of DNA as the genetic material. Just what is
that work? What must the genetic material be able to do?
There are at least three things.
- It Must Contain Information: DNA must have
somewhere in its molecular structure the information that
specifies what kind of organism its bearer becomes. This
is the information that directs cellular and organismal
processes. It must have the ability to determine that you
are a human and to determine your skin pigmentation, your
hair color, and your susceptibility or resistance to
various diseases (among countless other traits). From the
time that scientists conceded that DNA was the genetic
material, it was their working hypothesis (which turned
out to be true) that this information was in the sequence
of DNA's nitrogen bases.
- It Must Be Capable of Replicating Itself:
The information stored in a DNA molecule must be
copy-able. A DNA molecule must be capable of replicating,
thus producing two molecules with an identical base
sequence. That is the topic of this unit.
- It Must Be Capable of Determining Traits:
The information coded in the form of a base sequence must
have meaning that can be decoded. The sequence of bases
must somehow be capable of directing the cellular
activities within the organism. That is, it must be
capable of determining what kind of cell, tissue, organ,
and organisms develops. It must be capable of determining
traits. We will see that this process occurs by DNA
directing which proteins (including the all-important
enzymes) the cell makes.
- It Must Do Some Other Things: The three
roles of the genetic material above are absolutely
essential, but actually it also must be capable of other
functions, such as undergoing chemical and physical
changes (mutation) and break apart and joining with other
DNA molecules, as occurs during crossing over
(recombination). We will also take up these topics later.
|
- DNA Replication: Watson and Crick proposed a
simple model of DNA replication whereby the hydrogen bonds
holding the two strands of a DNA molecule are broken and the
two strands come apart (unwind). (This process is called
denaturation and will occur when DNA is heated to about 95
degrees C. The opposite process is annealing or
hybridization and occurs when denatured DNA is slowly
cooled.) Each single-stranded molecule can then serve as the
template for the synthesis of a new strand. The new strand
has a base sequence that is complementary to that of the
template (following A-T, G-C specific base pairing). This
model of replication is the semiconservative model of DNA
replication. (Each "new" DNA double helix is actually
composed of one newly-made strand and one "old" strand. That
is, the original molecule is half conserved in the new
molecule.)
|
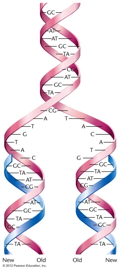 |
- The Proof of Semiconservative DNA Replication:
If DNA replication were not semiconservative, the other
main possibility was that it was conservative, where an
entirely newly-made double helix is made from the original
helix. (A dispersive model was also proposed.) The proof
that Watson and Crick's idea was right first came in
studying E. coli DNA replication.
|
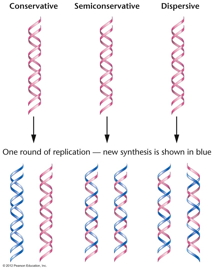 |
- Meselson and Stahl's Experiment in a
Prokaryote: In 1958, Meselson and Stahl
performed an experiment with E.
coli replication showing it to be
semiconservative.
|
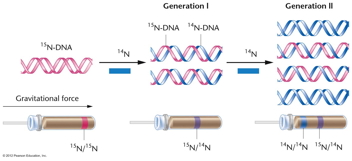 |
- Taylor, Woods, and Hughes' Experiment in a
Eukaryote: Taylor, Woods, and Hughes showed
that DNA replication is semiconservative in cells of the
fava bean plant using the technique of autoradiography.
|
- The Origin of Replication and the Replication
Fork: DNA replication occurs at a branch point
called a replication fork. Replication begins at a site
called the replication origin, which involves specific DNA
sequences in both prokaryotes and eukaryotes.
|
- Prokaryotes: Cairns demonstrated
that E. coli's chromosome has one
replication origin. That is, the entire circular
chromosome is one replicon. Replication from that origin
proceeds bidirectionally until the two replication forks
meet on the opposite side of the chromosome.
|
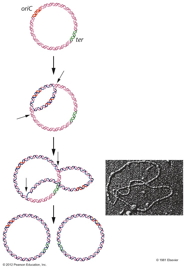 |
- Eukaryotes: Eukaryotic replication
begins at numerous replication origins with mammals
having about 25,000 origins across the genome.
Replication is bidirectional from each origin.
|
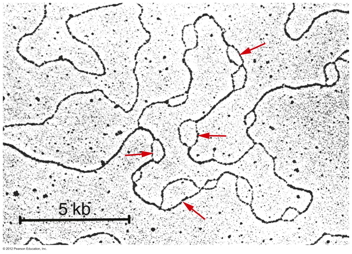 |
- The Enzymes of DNA Replication: There are
a number of enzymes that are required for replication of
DNA in vivo.
(Video showing how all of
the enzymes below may work together to replicate DNA)
|
- The DNA
Polymerases: The main class
of enzymes needed for DNA replication are the DNA
polymerases. These enzymes can add a nucleotide to the
3' OH of a primer as directed by the template strand
(base pairing). They use the deoxynucleoside
triphosphates (dATP, dGTP, dCTP, dTTP) with
pyrophosphate being cleaved off during the synthesis
reaction. A phosphodiester bond is formed and the 3' end
of the primer is extended (primer extension).
- Prokaryotes: The first DNA
polymerase discovered was by Arthur Kornberg (1957) in
E. coli and became known as DNA
polymerase I. Since DNA polymerase I mutants
could still grow and replicate their DNA, it was
realized that this must not be the main replication
enzyme (it is primarily a repair enzyme).
Subsequently, DNA
polymerase II and III were discovered (and
more). DNA polymerase III turns out to be the
main in vivo replication enzyme.
- Eukaryotes: Eukaryotes
have a number of DNA polymerases with polymerase δ
primarily responsible for in
vivo nuclear DNA replication.
|
- DNA
Helicase: This
enzyme works at the replication fork to break the
hydrogen bonds and thus unwind the DNA.
- SSBs: Since single-stranded DNA
collapses on itself, proteins called single-strand
binding proteins bind to the denatured single strands
maintaining the strand in linear form.
- A Problem (Solved by Topoisomerases like
Gyrase): The unwinding of the helix creates a
problem, since unwinding requires the rotation of the
double helix. The enzyme called topoisomerases solves
this problem by allowing rotation. (Topoisomerase
video)
- Another
Problem (Solved by the Enzyme Primase): The
fact
that
DNA synthesis only occurs in the 5' to 3' direction
creates another problem. Replication of one of the
strands can proceed without problem, but the
antiparallel nature of DNA means that replication
must proceed on the other strand in the opposite
direction. However, on that strand, there is no primer
to attach a nucleotide to (which is the only thing DNA
polymerase can do).
|
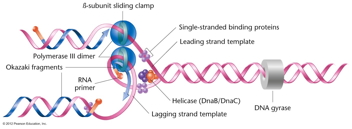
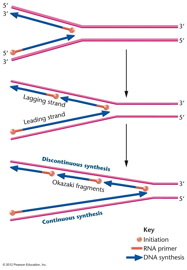
|
- Okazaki Fragments, Discontinuous (Lagging
Strand) and Continuous (Leading Strand) DNA
Replication: This problem was
initially solved by Okazaki who discovered that DNA
synthesis is continuous on one strand but
discontinuous on the other. That is, he discovered
that on the discontinuous strand, first short pieces
(Okazaki fragments, 1000 - 3000 bp) are made, then
joined together. Therefore, while the overall
direction of DNA synthesis on the discontinuous strand
is 3' to 5', the actual DNA synthesis on a molecular
level is occurring 5' to 3'. (Continuous strand
replication is also called leading strand replication
and discontinuous strand replication is called lagging
strand replication.)
|
- 5' ---> 3' Exonuclease Activity:
An enzyme must remove the RNA primer. This is
accomplished by some enzyme that has 5' ---> 3'
exonuclease* activity. The exonucleases involved in
removing the RNA primer removes one nucleotide at at
time from the 5' end. In E. coli, DNA
polymerase I (which has both 5' ---> 3' and 3'
---> 5' exonuclease activity in addition to its
polymerase activity) removes the RNA primer and
simultaneously synthesizes new DNA to replace it. In
eukaryotes, a special exonuclease called RNase H appears
to work along with other exonucleases to remove the RNA
primer in the 5' to 3' directions
- DNA Ligase: The nick left
between two Okazaki fragments must be sealed. DNA ligase
joins the two Okazaki fragments.
DNA
replication animations and videos:
|
- Still Another Problem (Solved by Eukaryotic Telomerase): The end of a linear
chromosome is called a telomere. Its replication problem
solved by an enzyme called telomerase.
Twins,
Space, and Telomeres: In a NASA study
where one twin was on earth and the other in space,
research has shown that
- "...the telomeres--the regions at the ends of
chromosomes--in Scott Kelly's white blood cells got
longer during the mission. Telomeres help protect
chromosomes from deterioration, and they get shorter
over the decades as people age.
Scott's telomere lengthening "could be linked to
increased exercise and reduced caloric intake during
the mission," NASA officials wrote in a description
of the newly announced findings.
"However, upon his return to Earth, they began to
shorten again," the officials added. "Interestingly,
telomerase activity (the enzyme that repairs the
telomeres and lengthens them) increased in both
twins in November, which may be related to a
significant, stressful family event happening around
that time." (http://www.space.com/35527-nasa-astronaut-twins-study-early-results.html)
|
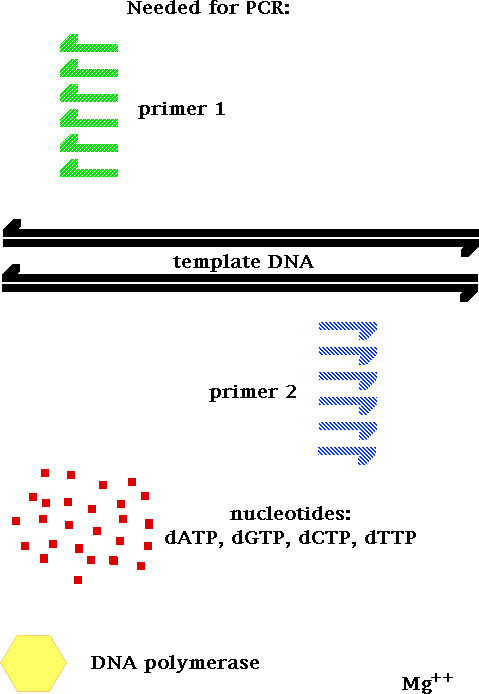 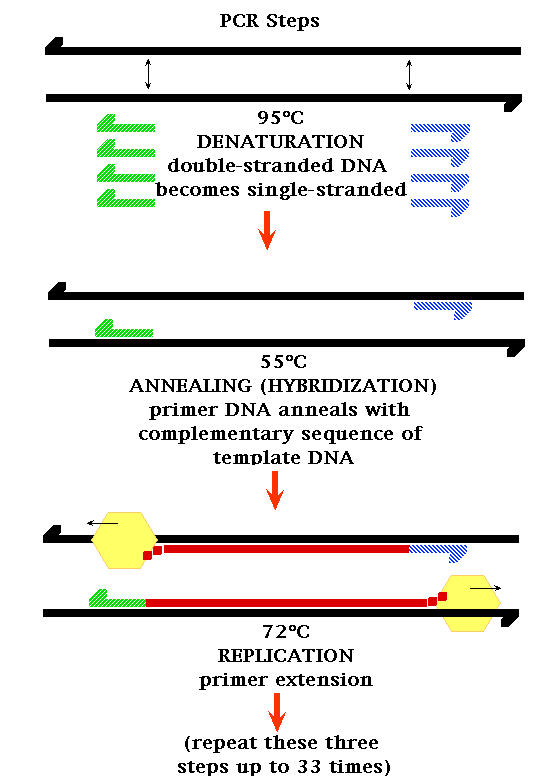
|
Things I
Learned at the Movies:
At least one of a pair of identical twins is born evil.
|