When
you have mutants you're better off that when you don't.
--Salvador E. Luria
A mutation is defined as any permanent change to the genetic
material. Mutation can be classified according to several
criteria. (There are also non-permanent changes to the genetic
material called epigenetic changes, like methylation of DNA
bases. See "What is Epigenetics" below.)
- Origin of the Mutation:
Mutations can be either spontaneous or induced.
- Spontaneous Mutations: Mutations occur
naturally, but at a very low rate. We will explore the
chemical nature of some of these spontaneous mutations
later. The rate for spontaneous mutations varies from
organisms to organism (10-4 to
10-8).
- Induced Mutations and Mutagens: Mutations
can be induced by various outside factors, called
mutagens.
- Chemical
Mutagens: Numerous chemicals can alter
DNA by reacting with it or creating other molecules that
react with DNA. Again, we will study some examples
later.
- Mutagenic
Radiation: Certain types of radiation
damage DNA.
- Ionizing Radiation: Some
radiation creates radicals or ion pairs as it passes
through tissue. These are very reactive and can
chemically modify DNA.
- X
rays: X rays are an
example of ionizing radiation. In 1927, H. J. Muller
first showed that X rays cause mutations.
- Others:
γ rays, β and α
particles, neutrons are other examples of ionizing
(therefore mutagenic) radiation.
- Ultraviolet
Radiation:
Although some UV can cause ionization, it is usually
mutagenic due to its ability to create covalent bonds
between adjacent thymine bases in DNA (thymine dimers,
more later).
- Site
of the Mutation:
In multicellular organisms, mutations can be classified as
either germ-line mutations or somatic mutations:
- Germ-Line Mutations: These are mutations
in gametes or cells destined to become gametes. They can
be passed along to future generations.
- Somatic Mutations: There are mutations in
the somatic cells and cannot be passed along.
- Carcinogenesis
and Mutagenesis: One class of somatic
mutations are those that turn a normal gene into a
cancer gene (oncogene). We will look at this process
briefly later in this course.
- Chromosome
Mutation vs. Gene Mutation:
Mutations can be classified as either chromosome mutations
or gene mutations.
- Chromosome Mutation: These mutations
change the chromosome number or chromosome structure, but
may not have any detectable effect on the individual
genes. Rather, the dosage and/or locus of a gene is
changed.
|
- Changes in
Chromosome Number: Many chromosome
mutations change the number of chromosomes.
- Euploidy:
This is the addition or subtraction of a whole haploid
set (genome).
- Haploidy:
This is the 1n condition in an organism that is
normally 2n and is very rare.
|
|
- Polyploidy:
This is the gain of one or more genomes (3n, 4n, 5n,
etc.). Polyploidy is common in many plants and some
lower animals. It may sexual or asexual.
|
- Autopolyploidy:
Autopolyploidy involves the gaining of a duplicate
genomes from the same species. This may occur
spontaneously or may be induced by microtubule
blockers like colchicine.
- Allopolyploidy:
Allopolyploidy is polyploidy by adding genomes of
different species.
|
- Aneuploidy
and Nondisjunction:
Aneuploidy is gaining or losing single (or several)
chromosomes. It is the main cause of spontaneous
abortions (35% of all) and is the main cause of mental
retardation. Aneuploidies are also extremely common in
cancer tumors (causative or not?). Aneuploidy most
commonly occurs by a mistake of meiosis called
nondisjunction, which usually occurs at anaphase I.
This is the failure of homologous chromosomes to
segregate.
|
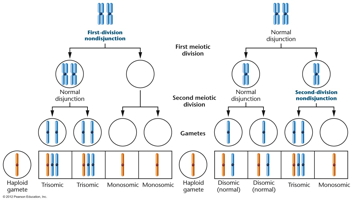 |
- Trisomy:
A trisomic individual has gained one
chromosome.
- Down
Syndrome:
Also called trisomy 21, this is a common
aneuploidy in humans. It usually occurs by
nondisjunction in oogenesis, with the rate
increasing with maternal age (average frequency =
1/700; age 25 = 1/1400; age 35 = 1/350; age 40 =
1/100).
|
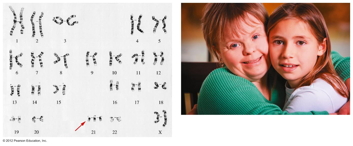
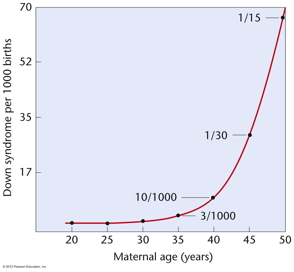
|
- Others:
There are other human trisomies that are not as
common as Down Syndrome, including trisomy 18
(Edwards Syndrome) (1/8,000) and trisomy 13
(1/20,000).
|
- Monosomy:
This is the loss of a single chromosome.
- Aneuploidy
of Sex Chromosomes:
These are common aneuploidies of sex chromosomes in
humans.
- Klinefelter Syndrome:
In humans, an XXY male individual has this
syndrome (1/500). (Other rare Klinefelter
individuals may be XXXY, XXYY, XXXXY, or even
XXXXXY. They have more severe symptoms.)
- Turner Syndrome:
In humans, an XO female has this syndrome
(1/2,500). Other Turner individuals may be missing
only a portion of the X chromosome. (See NIH
info site)
- Others: XXX, XYY and
other aneuploidies are possible.
- A Hypothesis for the
Mechanisms of Aneuploidy:
Aneuploidy may produces a plethora of effects but it
has been postulated from work originally in yeast
(confirmed in mammals) that aneuploidy creates
stress on the proteasome mechanism of cells.
Proteasomes are cellular complexes that include
proteases (protein-destroying enzymes). These
destroy unneeded or damaged (misfolded) proteins.
Thereby they help regulate the relative
concentration of proteins, which is important in
assembling proteins with tertiary structure. Having
an extra chromosome increases the concentration of
proteins made by genes on that chromosome. This put
stress on the mechanism that keeps the relative
concentration of proteins correct (proteasomes). In
fact, it appears that the higher the ploidy, the
lesser the effect of adding a single chromosome. (A
1n yeast cell with and extra chromosome is more
defective than a 2n cell with an extra chromosome,
presumably because the relative concentration of
proteins is further off in the 1n cell.) Targeting
cells with aneuploidies with drugs presents a novel
way of attaching cancer cells, since aneuploidy is
common in tumors. (Amon, GSA 2012)
|
- Changes
in Chromosome Structure:
Chromosomal segment may change their copy number or
location.
- Duplication:
If a segment is duplicated, it can be in one of three
configurations.
- Tandem Duplications:
These have the duplicated segment in the original
order and next to it.
|
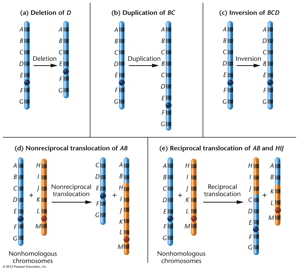 |
- Unequal Crossing
Over:
This mistake of meiosis I can cause both tandem
duplications and deletions. (Duplications and
deletions may occur by other mechanisms also.)
- Homologous
Unequal Crossing Over:
This is a secondary event that occurs as a
result of the presence of a previous tandem
duplication, as illustrated by the Bar
mutation in Drosophila.
- Nonhomologous
Unequal Crossing Over:
This is the original event that creates a tandem
duplication and deletion.
|
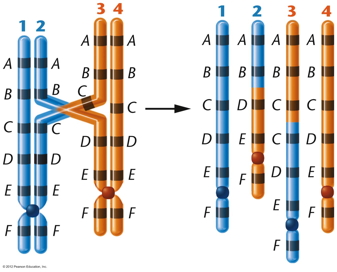
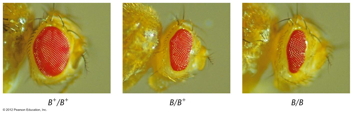
|
- Palindromes:
This is a repeat that is in reverse order compared
to the original segment.
- Displace
Duplications:
This is a duplication in which the duplicated
segment is not adjacent to the original segment.
- Human
Duplications:
- Hb Lepore
syndrome: Normal
adult hemoglobin (Hb) has two α globins and two β
globins: α2β2). The rare
genetic syndrome called Hb Lepore syndrome results
when a person has one mutant Lepore β Hb allele
and one normal β allele (that is, they are
heterozygotes). They are usually asymptomatic.
However, homozygotes for the Lepore β allele have
β-thalassemia. (β-thalaseemia is a general term
for severe anemia in which there is decreased
production of the β chain.) The Lepore allele most
likely arose by homologous unequal crossing over
between the δ and β globin genes on chromosome 11,
resulting in a δ/β fusion gene which codes for the
altered Hb called Hb Lepore , which is a
polypeptide that is a fusion of the amino end of
the δ polypeptide and the carboxyl end of the β
polypeptide. [Non-homologous unequal crossing over
is believed to have given rise to the δ allele in
the human evolutionary past. Homologous unequal
crossing over is believed to be the origin of the
δ/β fusion allele.]
- Huntington Disease: This is an autosomal dominant
trait (involving the HTT gene on
chromosome 4) with incomplete
penetrance that is due to a trinucleotide
repeat (CAG)n, encoding multiple glutamines in the
huntingtin protein (the protein coded for by the
mutant HTT allele). The normal number of
repeats is between 9 and 36. Persons with 36-39
repeats show the reduce penetrance describe in the
Mendel Revisited
outline (McNeil SM et al. 1997, Hum Mol Genet.
6:775). Juvenile-onset Huntington disease (onset
before age 20) is usually inherited from the
father and affected individuals have 60 or more
repeats. However, "a substantial proportion of the
variance in age of onset in Huntington disease is
due either to variation in genes other than HTT
or in the environment" (Wexler et al. 2004. Proc
Natl Acad Sci USA 101:3498). The function of
huntingtin in the cell is unknown..." as of 2012
(http://www.ncbi.nlm.nih.gov/pubmed/22482451), but
it is known to have its effect on brain cells.
- Fragile-X Syndrome: A
fairly common, X-linked dominant genetic disorder,
it also involves extra copies of a trinucleotide
repeat, like Huntington Disease. Read and know
explanation in this link of fragile-X. Know the
info under Description, Frequency, Genetic
Changes, and Inheritance Pattern:
https://ghr.nlm.nih.gov/condition/fragile-x-syndrome#inheritance
- Deletions:
Like tandem duplications, deletion (also called
deficiencies) can also be caused by unequal crossing
over (and other mechanisms). (Cri-du-chat,
first documented human deletion). Duplications and
deletions may be referred to as copy number variants
(CNVs).
|
- Inversions:
Inversions involve reversing the orientation of a
segment of a chromosome. Inversion may be paracentric
or pericentric. Inversion heterozygotes have problems
making functional gametes due to crossing over in the
inverted segment.
|
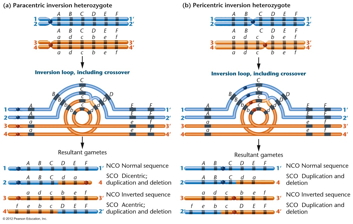
|
- Translocations: Translocations
move a segment to a non-homologous chromosome. Just as
with inversion heterozygotes, reciprocal
translocation heterozygotes have reduced fertility.
|
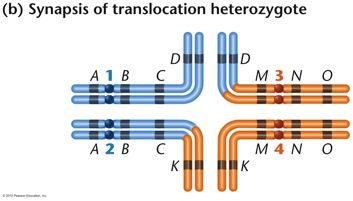 |
- Robertsonian
Translocation (Robertsonian Fusion): A
nonhomologous exchange between two acrocentric or
telocentric chromosomes can result in a fused
(metacentric) chromosome. (Reference:
mechanisms may result in two closely adjacent
centromeres and one acentric fragment, rather than
as illustrated to the right. Here
is a more recent article proposing how Robertsonian
translocations between two telocentric
chromosomes may occur.)
|
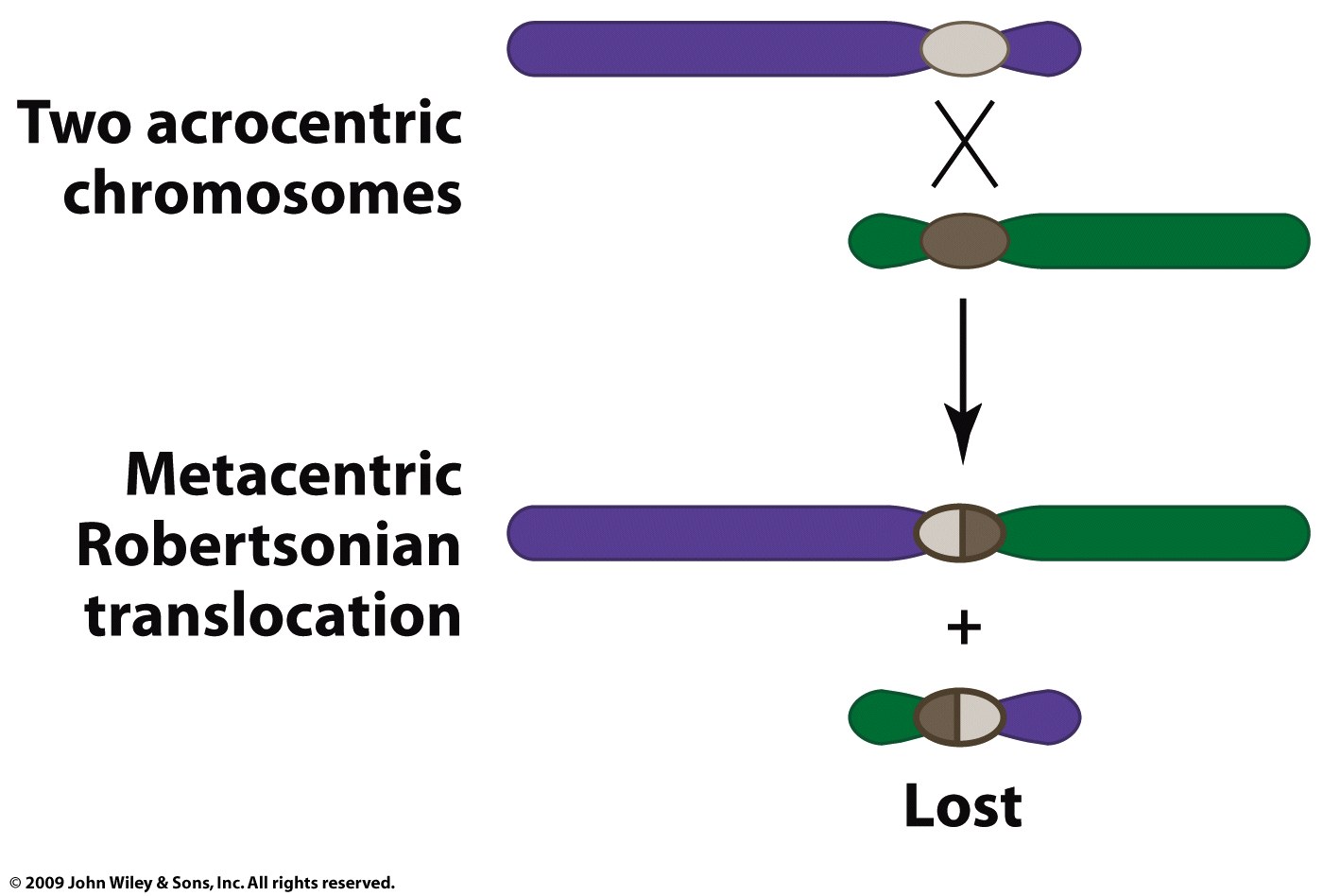 |
- Karyotyping and
Chromosome Mutations:
Karyotyping
makes it possible to detect chromosome mutations. The
human karyotype divides the chromosome into groups A, B,
C, D, E, F, G, and sex chromosomes. (Arrange in size
order, large to small; A group (3 pairs) = metacentric
and submetacentric, B (2 pairs) group = submetacentric,
C group (7 pairs) = metacentric, D group (3 pairs) =
acrocentric, E group (3 pairs) = metacentric, F group (2
pairs), G group (2 pairs) = acrocentric, X chromosome =
resemble a C group chromosome, Y chromosome = resembles
a G group chromosome.)
- Gene Mutations: (Next outline)
What Is Epigenetics? (from:
http://www.zymoresearch.com/learning-center/epigenetics/what-is-epigenetics)
"Epigenetics" refers to covalent modification of DNA,
protein, or RNA, resulting in changes to the function and/or
regulation of these molecules, without altering their primary
sequences. In some cases, epigenetic modifications are stable
and passed on to future generations, but in other instances they
are dynamic and change in response to environmental stimuli.
Nearly every aspect of biology is influenced by epigenetics,
making it one of the most important fields in science.
Epigenetics and Me
Why do some foods cause health problems and others make
us healthy? How does stress impact our long-term well-being? Why
is it that the older we get, the more likely it is that
age-related illness will strike us? Unlocking the secrets behind
these and other questions has the potential to revolutionize
life as we know it. The emerging field of epigenetics is aiming
to do just that.
The importance of nature versus nurture has long been
disputed. It cannot be denied that environment greatly
influences how a child grows and develops, nor can it be denied
that our DNA is the blueprint that makes us who we are.
Epigenetics merges these two seemingly contradictory lines of
thought to explain how environmental factors cause physical
modifications to DNA and its associated structures, which result
in altered functions.
The most commonly known epigenetic modification is DNA
methylation. Although many technologies have been developed in
the past to characterize genomic DNA methylation, none of them
has been able to efficiently determine DNA methylation patterns
on a genomic scale. Until now.
More on Epigenetics
Many cellular processes, including gene expression and
DNA replication, are often regulated by mechanisms that fall
into the category of "classical genetics." This generally means
that they are controlled by elements such as promoters,
enhancers, or binding sites for repressor proteins, that are
present or absent in the DNA sequence. An example of this
type of regulation is the control of expression of a cellular
oncogene. In normal (non-cancer) cells, this gene would
not be expressed. However, in a cancer cell, this gene could
have acquired a mutation, which is a change to the DNA sequence,
that allows the oncogene to be expressed, and thus can
contribute to the progression of cancer.
In addition to the regulatory mechanisms of classical
genetics, nearly all cellular processes can also be regulated by
epigenetic mechanisms. Epigenetic mechanisms can be just
as important to biological events as genetic mechanisms, and can
also result in stable and heritable changes. However, the big
difference between genetic and epigenetic regulation is that
epigenetic mechanisms do not involve a change to the DNA
sequence, whereas genetic mechanisms involve the primary DNA
sequence and changes or mutations to this sequence.
Epigenetic regulation involves the modification of DNA and the
proteins associated with DNA, which results in changes to the
conformation of DNA and accessibility of other factors to DNA,
without a change to the sequence of the DNA.
The Greek prefix "epi" means "on top of" or "over", so
the term "Epigenetics" literally describes regulation at a level
above, or in addition to, those of genetic mechanisms. Common
types of epigenetic regulation are DNA methylation and
hydroxymethylation, histone modification, chromatin remodeling,
and regulation by small and large non-coding RNAs. The field of
epigenetics was given its name and a vague definition only ~50
years ago, but is now a dynamic and rapidly expanding
discipline, challenging and revising traditional paradigms of
inheritance.
Through epigenetics, the classic works of Charles Darwin,
Gregor Mendel, and Jean-Baptiste Lamarck and others are now seen
in different ways. As more factors influencing heredity are
discovered, today's scientists are using epigenetics to decipher
the roles of DNA, RNA, proteins, and environment in inheritance.
The future of epigenetics will reveal the complexities of
cellular differentiation, embryology, the regulation of gene
expression, aging, cancer, and other diseases.
|
Things I Learned at the
Movies:
Radiation causes interesting
mutations — not to your future children, but to you, right
then and there.
|